Enceladus’ subsurface saline ocean has long made it a compelling location to search for signs of extraterrestrial life in our solar system. Cryovolcanic plumes are important targets for sampling the chemical content of these oceans – specifically, to search for organic molecules that can provide valuable insight into primordial sources of organic matter and to investigate whether organic synthesis is possible in an environment such as Enceladus.
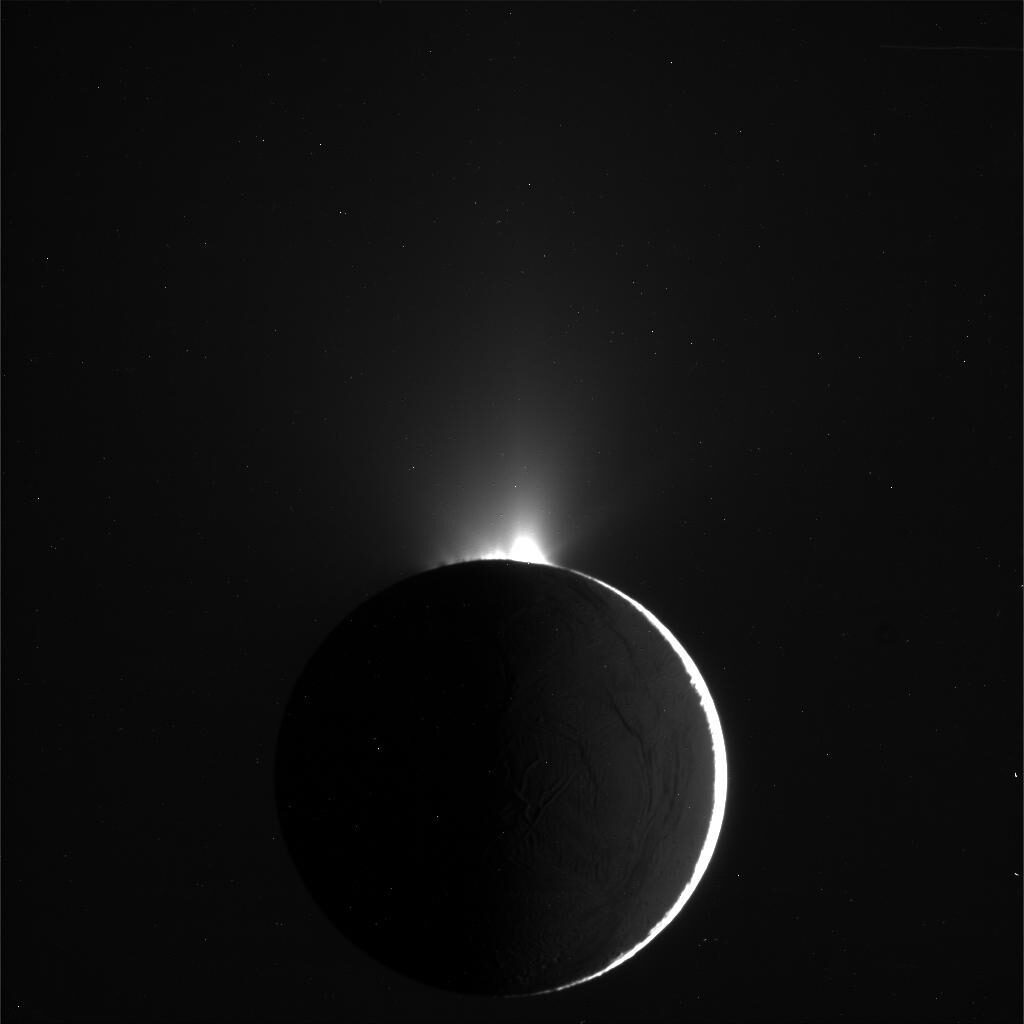
Image Credit: NASA/JPL/Space Science Institute
Source
Data from the Cassini mission suggests that the plumes contain trace amounts of low and high mass organic material. These could be collected by fly-by mission, which can be performed with a smaller spacecraft, at a lower cost, and with lower risk than a landed mission. However, a more effective means of capturing material from the plumes is needed for in situ analysis of Enceladus’ plumes. Our hypervelocity impact experiments use a light gas gun and organic ice particle simulants to test whether captured particles would remain intact after impact, and to quantify the effects of different particle sizes and impact velocities on capture efficiency.
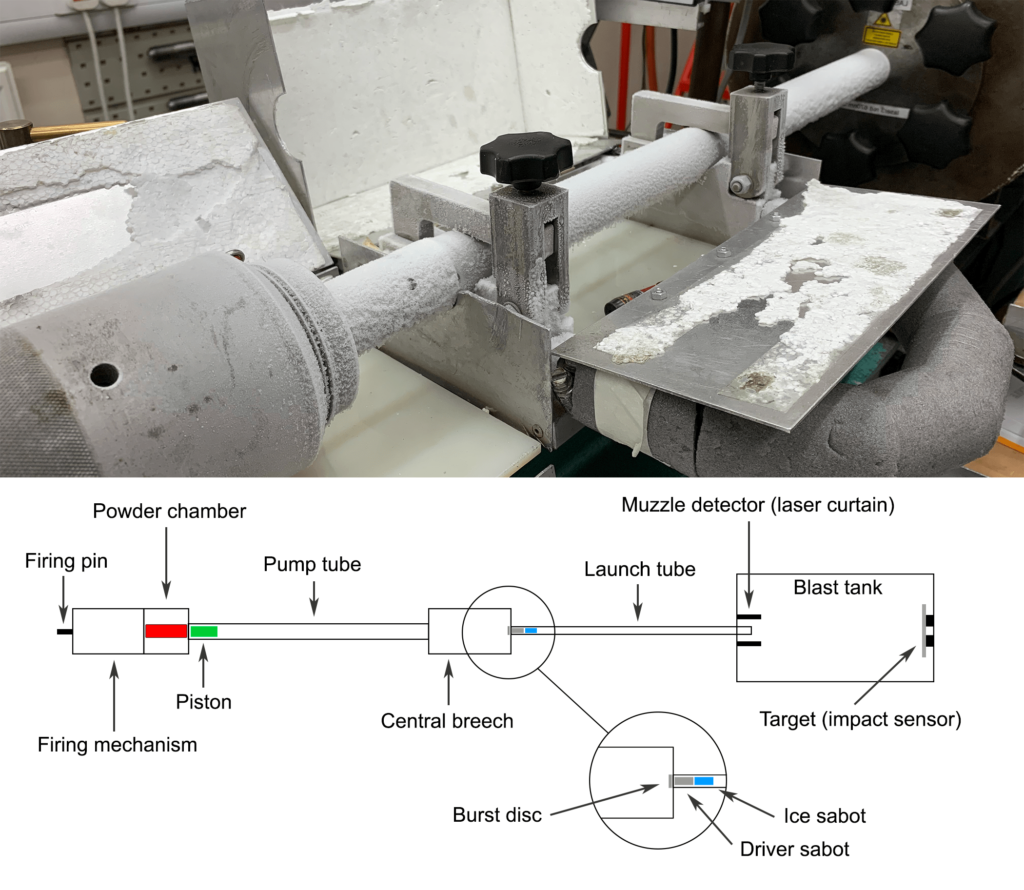
The light gas gun was first used to accelerate organic ice-particle simulants into soft inert metal targets at velocities comparable to the plumes on Enceladus. The material used for organic capture needs to be easily cleaned and accommodate dissolution of particles for analysis. Different metals were used, and the craters left by particles were analyzed for retention of organic material, as well as molecular survival.
The first experiment was conducted using Poly(methyl methacrylate) (PMMA) particles to simulate the capture of ice with known particle size. PMMA was chosen because of its similar mechanical properties to ice, and because its Raman signature remains the same even if the material melts. This allowed us to confirm that the organic chemical structure is still intact after impact. PMMA also has a low melting point (160 C) relative to its impact energy, making it possible to investigate the phase changes expected in ice impacts.
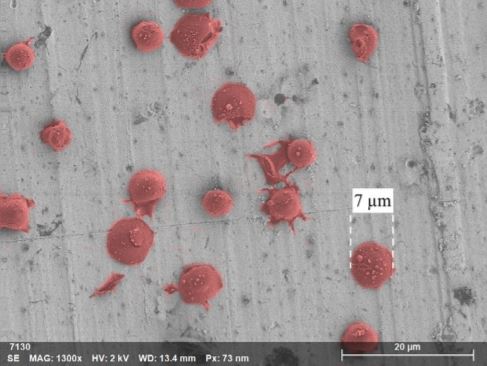
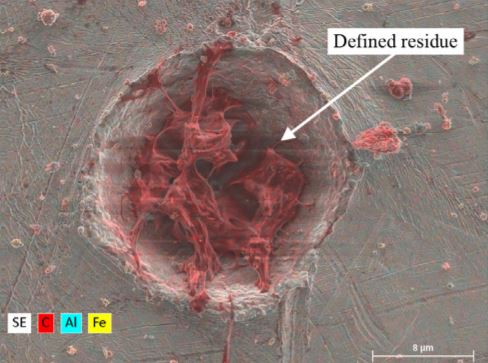
Well-defined stringy residue is highlighted in red.
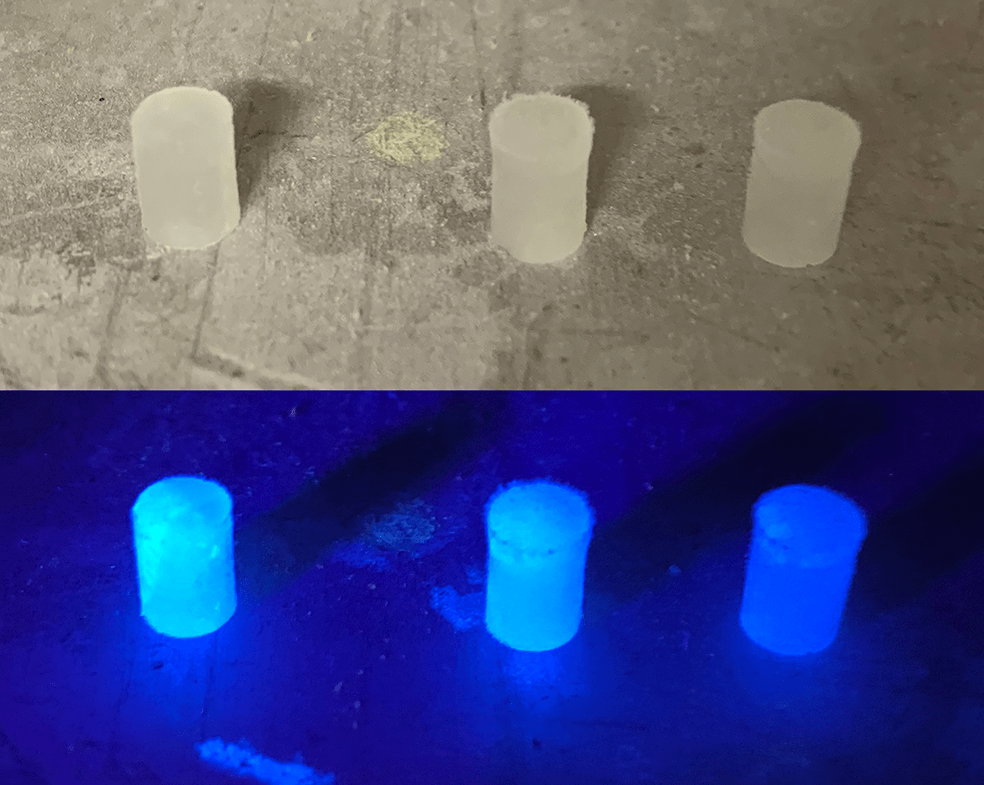
The second set of experiments used ice doped with fluorescent dye, sodium chloride, and a pH buffer. In this case, the ice shatters into a range of small particles on impact, with the diameter of the ice fragments derived from PMMA calibration plots. The dye enabled us to observe both the capture and survival of the material. The craters were categorized to quantify the capture efficiency with respect to target material, impact velocity, and particle size. It should be noted that the bonds in the fluorescent dye break more easily than those in the amino acids we are looking for on Enceladus, so this is a conservative approach.
The results clearly demonstrate that an indium capture surface can provide successful capture of intact organic molecules entrained in high-velocity (1–3 km/s) ice particles and that capture efficiencies of ~10% can be expected for a large number of impacts, and as high as ~50% for individual impacts. The optimal velocity was between 1–2 km/s, where high capture efficiencies were observed in over 25% of the craters.
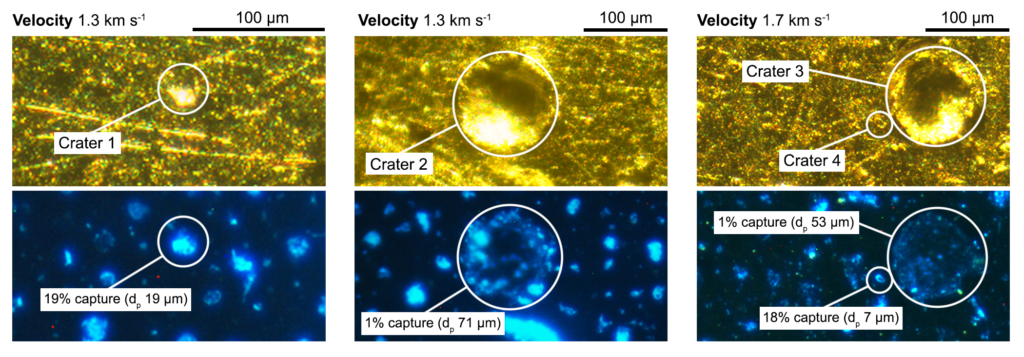
Fluorescing Pacific Blue™ residue is observed in the circled craters and the corresponding particle diameters and organic capture efficiencies are shown
Our experiments are the most realistic and the only quantitative evaluation of the feasibility of organic capture and analysis from the Enceladus plume. Since accumulative capture on the capture surface can be achieved via multiple plume transits EOA can utilize a large ice sample when deployed as an Enceladus orbiter. This is an important step forward in investigating the possibility of organic synthesis on Enceladus, and experiments of this type can ultimately enable predictions of the measurement capabilities of various scientific instruments.